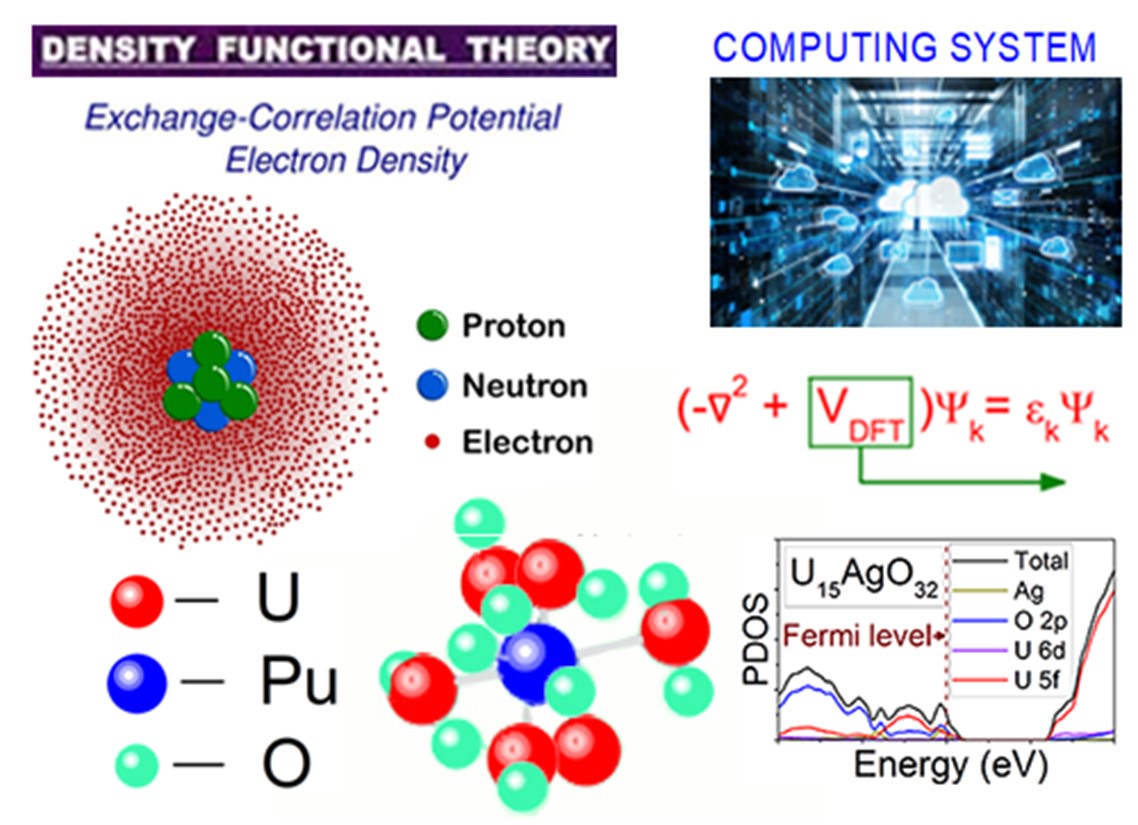
First principle study of the physical properties of actinide oxides. A review
Abstract
Reprocessing spent nuclear fuel creates the conditions for converting nuclear energy to a clean and sustainable energy. Thermal conductors in reactors are molten salts, the safe use of which is based on an accurate knowledge of their thermophysical properties. Due to the difficulty of experimentally measuring these properties, computational methods are coming to the fore. The main component of SNF is uranium dioxide, the change in the structure, energy and electronic properties of which when oxygen is removed from the system and plutonium is added to it was studied using DFT + U calculations. The influence of noble metals present in spent fuel on the process of direct reduction of irradiated nuclear fuel is considered. This work also examines the effects of doping thorium with uranium when Th-U fuel is used in a nuclear reactor. The paper presents data on the calculation of heat capacity, thermal conductivity, elastic moduli and phase transitions occurring in UO2 at high pressures. The thermodynamic stability of actinide oxides is discussed in detail. The ways of improving DFT calculations to increase the accuracy of determining the properties of these nuclear materials are analyzed.
Keywords
Full Text:
PDFReferences
Ripani M, Energy from nuclear fission, Eur. Phys. J. Conf., 268 (2022) 00010. https://doi.org/10.1051/epjconf/202226800010
Galashev AY, Recovery of actinides and fission products from spent nuclear fuel via electrolytic reduction: Thematic overview, Int. J. Energy Res., 46(5) (2021) 3891–3905. https://doi.org/10.1002/er.7458
Galashev AY, Processing of fast neutron reactor fuel by electrorefining: Thematic overview, Int. J. Energy Res., 45 (2020) 11459–11478. https://doi.org/10.1002/er.6267
Van Winckel S, Alvarez-Sarandes R, Purrov DS, Aldave de las Heras L, Assay data of spent nuclear fuel: the lab-work behind the numbers, Front. Energy Res., 11 (2023) 1168460. https://doi.org/10.3389/fenrg.2023.1168460
Pegg JT, Aparicio-Angles X, Storr M, de Leeuw NH, DFT+U study of the structures and properties of the actinide dioxides, J. Nucl. Mater., 492 (2017) 269–278. https://doi.org/10.1016/j.jnucmat.2017.05.025
Wang B-T, Shi H, Li W, Zhang P, First-principles LDA+U and GGA+U study of neptunium dioxide, Phys. Rev. B, 81 (2010) 045119. https://doi.org/10.1103/PhysRevB.81.045119
Moten SA, Atta-Fynn R, Ray AK, Muhammad N., Huda MN, Size effects on the electronic and magnetic properties of PuO2 (111) surface, J. Nucl. Mater., 468 (2016) 37–45. https://doi.org/10.1016/j.jnucmat.2015.11.009
Gueneau C, Dupin N, Sundman B, Martial C, et al., Thermodynamic modelling of advanced oxide and carbide nuclear fuels: Description of the U–Pu–O–C systems, J. Nucl. Mater., 419 (2011) 145–167. https://doi.org/10.1016/j.jnucmat.2011.07.033
Dennett CA, Poudel N, Simmons PJ, Tiwari A, et al., Towards actinide heterostructure synthesis and science, Nature Commun., 13 (2022) 2221. https://doi.org/10.1038/s41467-022-29817-0
Suzuki M-T, Magnani N, Oppeneer PM, Microscopic theory of the insulating electronic ground states of the actinide dioxides AnO2 (An = U, Np, Pu, Am, and Cm), Phys. Rev. B, 88 (2013) 195146. https://doi.org/10.1103/PhysRevB.88.195146
Shi H, Chu M, Zhang P, Optical properties of UO2 and PuO2, J. Nucl. Mater., 400(2) (2010) 151–156. https://doi.org/10.1016/j.jnucmat.2010.02.024
Wang H, Konashi K, LDA + U study of Pu and PuO2 on ground state with spin–orbital coupling, J. Alloys Compounds, 533 (2012) 53–57. https://doi.org/10.1016/j.jallcom.2012.03.117
Noutack MST, Geneste G, Jomard G, Freyss M, Investigation of the bulk and point defect properties in uranium–plutonium mixed oxides (U,Pu)O2 using DFT+U: Effect of a low americium content, J. Appl. Phys., 131 (2022) 225106. https://doi.org/10.1063/5.0090000
Zhu J, Shi D, The electronic and mechanical properties of (U, Th)O2 compounds: a first-principles study, RSC Adv., 13 (2023) 7206–7211. https://doi.org/10.1039/D3RA00018D
Dorado B, Garcia P, First-principles DFT + U modeling of actinide-based alloys: Application to paramagnetic phasesof UO2 and (U,Pu) mixed oxides, Phys. Rev. B, 87 (2013) 195139. https://doi.org/10.1103/PhysRevB.87.195139
Burakovsky L, Ramsey SD, Baty RS, Ambient melting behavior of stoichiometric uranium-plutonium mixed oxide fuel, Appl. Sci., 13 (2023) 6303. https://doi.org/10.3390/app13106303
Fouquet-Métivier P, Martin PM, Manara D, Dardenne K, et al., Investigation of the solid/liquid phase transitions in the U–Pu–O system, Calphad, 80 (2023) 102523. https://doi.org/10.1016/j.calphad.2022.102523
Sheykhi S, Payami M, First-principles study of UO2 lattice thermal-conductivity: A simple description, Iran J. Sci. Technol., 44(8) (2020) 2585–1593. https://doi.org/10.1007/s40995-020-00927-y
Mir SH, Jha PC, Islam MS, Banerjee A, et al., Static and dynamical properties of heavy actinide monopnictides of lutetium, Sci. Rep., 6 (2016) 29309. https://doi.org/10.1038/srep29309
Burakovsky L, Ramsey SD, Baty RS, Ambient melting behavior of stoichiometric uranium oxides, Front. Nucl. Eng., 2 (2024) 1215418. https://doi.org/10.3389/fnuen.2023.1215418
Vorob’ev AS, Galashev AY, Zaikov YuP, Quantum mechanical study of the influence of noble metals on the process of reduction of uranium oxides, Nucl. Eng. Technol., (2024). In press. https://doi.org/10.1016/j.net.2024.09.020
Galashev A, Abramova K, Vorob’ev A, Rakhmanova O, Zaikov Yu, Modeling the UO2 reduction process, Electrochem. Mater. Technol., 2 (2023) 20232017. http://dx.doi.org/10.15826/elmattech.2023.2.017
Dudarev SL, Botton GA, Savrasov SY, Humphreys C, et al., Electron-energy-loss spectra and the structural stability of nickel oxide: An LSDA+U study, Phys. Rev. B, 57(3) (1998) 1505–1509. https://doi.org/10.1103/PhysRevB.57.1505
Monkhorst HJ, Pack JD, Special points for Brillouin-zone integrations, Phys. Rev. B, 13(12) (1976) 5188–5192. https://doi.org/10.1103/PhysRevB.13.5188
Perdew JP, Zunger A, Self-interaction correction to density-functional approximations for many-electron systems, Phys. Rev. B, 23(10) (1981) 5048–5079. https://doi.org/10.1103/PhysRevB.23.5048
Shields AE, Santos-Carballal D, de Leeuw NH, A density functional theory study of uranium-doped thoria and uranium adatoms on the major surfaces of thorium dioxide, J. Nucl. Mater., 473 (2016) 99–111. https://doi.org/10.1016/j.jnucmat.2016.02.009
Perdew JP, Burke K, Ernzerhof M, Generalized gradient approximation made simple, Phys. Rev. Lett., 77 (1996) 3865–3868. https://doi.org/10.1103/PhysRevB.23.5048
Kresse G, Joubert D, From ultrasoft pseudopotentials to the projector augmented-wave method, Phys. Rev. B, 59 (1999) 1758–1775. http://dx.doi.org/10.1103/PhysRevB.59.1758
Blochl PE, Projector augmented-wave method, Phys. Rev. B, 50 (1994) 17953–17979. https://doi.org/10.1103/PhysRevB.50.17953
Galashev AY, Vorob’ev AS, Zaikov YuP, Quantum-mechanical study of the electronic properties of UxPuyOz compounds formed during the recovery of spent nuclear fuel, J. Serb. Chem. Soc., 88(11) (2023) 1125–1134. https://doi.org/10.2298/JSC230213038G
Abdulaziz R, Brown L, Inman D, Sharrad CA, et al., Electrochemical reduction of UO2 to U in LiCl-KCl molten salt eutectic using the fluidized cathode process, J. Electrochem. Soc., 164(8) (2017) H5280. https://doi.org/10.1149/2.0421708jes
Jamal GEl, Gouder T, Eloirdi R, Jonsson M, Idriss H, Monitoring the reduction of UO3 thin film by hydrogen atoms using valence-level spectroscopy: correlating the U5f1 signal to surface hydroxyls, Front. Fuels, 1 (2023) 1303890. https://doi.org/10.3389/ffuel.2023.1303890
Shundalaua MB, Zajogina AP, Komiaka AI, SokolskyaAA, Umreiko DS, DFT modeling of the uranium trioxide vibration spectra characteristics, J. Spectrosc. Dyn., 2 (2012) 19. https://elib.bsu.by/bitstream/123456789/160888/2/110637239.pdf
Kaloni TP, Onder N, Pencer J, Torres E, DFT+U approach on the electronic and thermal properties of hypostoichiometric UO2, Ann. Nucl. Energy, 144 (2020) 107511. https://doi.org/10.1016/j.anucene.2020.107511
Dugan CL, Peterson GG, Mock A, Young C, et al., Electrical and material properties of hydrothermally grown single crystal (111) UO2, Eur. Phys. J. B, 91 (2018) 67. https://doi.org/10.1140/epjb/e2018-80489-x
Choi E-Y, Kim J-K, Im H-S, Choi I-K, et al., Effect of the UO2 form on the electrochemical reduction rate in a LiCl–Li2O molten salt, J. Nucl. Mater., 437 (2013) 178–187. https://doi.org/10.1016/j.jnucmat.2013.01.306
Hur J-M, Hong S-S, Lee H, Electrochemical reduction of UO2 to U in a LiCl–KCl–Li2O molten salt, J. Radioanal. Nucl. Chem., 295 (2013) 851–854. https://doi.org/10.1007/s10967-012-2258-0
Choi E-Y, Won C-Y, Cha J-S, Park W, et al., Electrochemical reduction of UO2 in LiCl–Li2O molten salt using porous and nonporous anode shrouds, J. Nucl. Mater., 444 (2014) 261–269. https://doi.org/10.1016/j.jnucmat.2013.09.061
Choi E-Y, Jeong SM, Electrochemical processing of spent nuclear fuels: An overview of oxide reduction in pyroprocessing technology, Prog. Nat. Sci.: Mater. Intern., 25(6) (2015) 572–582. https://doi.org/10.1016/j.pnsc.2015.11.001
Kim SW, Lee SK, Kang HW, Choi EY, et al., Electrochemical properties of noble metal anodes for electrolytic reduction of uranium oxide, J. Radioanal. Nucl. Chem., 311 (2017) 809–814. https://doi.org/10.1007/s10967-016-5107-8
Broczkowsk ME, Goldic JS, Noel J, Shoesmith DW, Influence of noble metal particles on redox reactions on uranium dioxide surfaces, Adv. Sci. Technol., 45 (2006) 1996–2003. https://doi.org/10.4028/www.scientific.net/AST.45.1996
Shishkin AV, Shishkin VYu, Pankratov AA, Burdina AA, Zaikov YuP, Electrochemical reduction of La2O3, Nd2O3, and CeO2 in LiCl-Li2O melt, Materials, 15(11) (2022) 3963. https://doi.org/10.3390/ma15113963
Shishkin AV, Shishkin VYu, Maslennikova AA, Salyulev A, et al., Electrochemical reduction of Pd-Nd2O3-CeO2 mixtures in the LiCl-Li2O melt, Processes, 11(6) (2023) 1599. https://doi.org/10.3390/pr11061599
Steinberg S, R. Dronskowski R, The crystal orbital Hamilton population (COHP) method as a tool to visualize and analyze chemical bonding in intermetallic compounds, Crystals, 8(5) (2018) 225. https://doi.org/10.3390/cryst8050225
Yang Y, Gao P, Li L, Pan X, et al., Electrochemical dynamics of nanoscale metallic inclusions in dielectrics., Nat. Commun., 5 (2014) 4232. https://doi.org/10.1038/ncomms5232
Merwin A, Phillips WC, Williamson MA, Willit JL, et al., Presence of Li clusters in molten LiCl-Li, Sci. Rep., 6 (2016) 25435. https://doi.org/10.1038/srep25435
Szpunar B, Szpunar J, Thoria ehancement of nuclear reactor safety, Phys. Int., 4(2) (2013) 110–119. https://doi.org/10.3844/pisp.2013.110.119
Liu B, Aidhy DS, Zhang Y, Weber WJ, Theoretical investigation of thermodynamicstability and mobility of the oxygen vacancyin ThO2–UO2 solid solutions, Phys. Chem. Chem. Phys., 16 (2014) 25461–25467. https://doi.org/10.1039/c4cp03660c
Kelly TD, Petrosky JC, Turner D, McClory JW, Mann JM, The unoccupied electronic structure characterization of hydrothermally grown ThO2 single crystals, Phys. Status. Solidi RRL, 8(3) (2014) 283–286. https://doi.org/10.1002/pssr.201308286
Konings RJM, Benes O, Kovacs A, Manara D, et al., The thermodynamic properties of the f-elements and their compounds. Part 2. The lanthanide and actinide oxides, J. Phys. Chem. Ref. Data, 43(1) (2014) 013101. https://doi.org/10.1063/1.4825256
Korotaev P, Yanilkin AV, Steels classification by machine learning and calphad methods, Calphad, 82(5) (2023) 102587. https://doi.org/10.1016/j.calphad.2023.102587
Wang B-T, Zhang P, Lizárraga R, Di Marco I, Eriksson O, Phonon spectrum, thermodynamic properties, and pressure-temperature phase diagram of uranium dioxide, Phys. Rev. B, 88 (2013) 104107. https://doi.org/10.1103/PhysRevB.88.104107
Wang R, Wang S, Wu X, Ab initio study of the thermodynamic properties of rare-earth-magnesium intermetallics MgRE (RE=Y, Dy, Pr, Tb), Physica Scripta, 83 (2011) 065707. https://doi.org/10.1088/0031-8949/83/06/065707
Sanati M, Albers R, Lookman T, Saxena A, Elastic constants, phonon density of states, and thermal properties of UO2, Phys. Rev. B, 84 (2011) 014116. https://doi.org/10.1103/PhysRevB.84.014116
Sharma Y, Paudel B, Huon A, Schneider MM, et al., Induced ferromagnetism in epitaxial uranium dioxide thin films, Adv. Sci., 9(33) (2022) 2203473. https://doi.org/10.1002/advs.202203473
Pegg JT, Shields AE, Storr MT, Wills AS, et al., Magnetic structure of UO2 and NpO2 by first-principle methods, Phys. Chem. Chem. Phys., 21 (2019) 760–771. https://doi.org/10.1039/C8CP03581D
Gao H, Li M, Yang Y, Zhang P, First-principles calculation of structural, magnetic and electronic properties of PuO2-H , 0≤x≤2, J. Alloys Compounds, 857 (2020) 157592. https://doi.org/10.1016/j.jallcom.2020.157592
Islam M, DFT and DFT+U Insights into the physical properties of UO2, J. Sci. Res., 15(3) (2023) 739–757. http://doi.org/10.3329/jsr.v15i3.64394
Torres E, Kaloni TP, Thermal conductivity and diffusion mechanisms of noble gases in uranium dioxide: A DFT+U study, J. Nucl. Mater., 521 (2019) 37–45. https://doi.org/10.1016/j.jnucmat.2019.04.040
Fink JK, Thermophysical properties of uranium dioxide., J. Nucl. Mater., 279(1) (2000) 1–18. https://doi.org/10.1016/S0022-3115(99)00273-1
Minamoto S, Kato M, Konashi K, Kawazoe Y, Calculations of thermodynamic properties of PuO2 by the first-principles and lattice vibration, J. Nucl. Mater., 385(1) (2009) 18–20. https://doi.org/10.1016/j.jnucmat.2008.10.024
Sobolev V, Modelling thermal properties of actinide dioxide fuels, J. Nucl. Mater., 344(1–3) (2005) 198–205. https://doi.org/10.1016/j.jnucmat.2005.04.042
Yang Y, Wang B, Zhang P, Electronic and mechanical properties of ordered (Pu, U) O2 compounds: A density functional theory +U study, J. Nucl. Mater., 433(1–3) (2013) 345–350. https://doi.org/10.1016/j.jnucmat.2012.10.027
Nye JF. Physical properties of crystals. Oxford University Press: Oxford; 1985. 352 p. ISBN 9780198511656
Cognini L, Pizzocri D, Barani T, Van Uffelen P, et al., Helium solubility in oxide nuclear fuel: Derivation of new correlations for Henry’s constant, Nucl. Eng. Design, 340 (2018) 240–244. https://doi.org/10.1016/j.jnucmat.2022.153777
Terez EI, Terez IE, Thermonuclear reaction as the main source of the Earth’s energy, Int. J. Astron. Astrophys., 3 (2013) 362–365. http://dx.doi.org/10.4236/ijaa.2013.33040
Greaux S, Gautron L, Andrault D, Bolfan N, et al., Structural characterization of natural UO2 at pressures up to 82 GPa and temperatures up to 2200 K, Am. Mineralogist, 93(7) (2008) 1090–1098. http://dx.doi.org/10.2138/am.2008.2735
Idiri M, Le Bihan T, Heathman S, Rebizant J, Behavior of actinide dioxides under pressure: UO2 and ThO2, Phys. Rev. B, 70 (2004) 14113. https://doi.org/10.1103/PhysRevB.70.014113
Tian X, Wang Y, Ge L, Dong W, et al., First principles calculation of UO2 polymorphs and phase transitions under compressive and tensile loading, Comput. Mater. Sci., 169 (2019) 109124. https://doi.org/10.1016/j.commatsci.2019.109124
Singh S, Sonvane Y, Nekrasov KA, Boyarchenkov AS, et al., Systematic investigation of electronic, mechanical and optical properties of UO2 at higher pressure: A DFT+U+SOC study, Solid State Sci., 132 (2022) 106968. https://doi.org/10.1016/j.solidstatesciences.2022.106968
Yu J, Devanathan R, Weber WJ, First-principles study of defects and phase transition in UO2, J. Phys. Condens. Mater., 21(43) (2005) 435401. https://doi.org/10.1088/0953-8984/21/43/435401
Yadigaroglu G, Nuclear reactors: Physics and materials, CHIMIA Int. J. Chem., 59(12) (2005) 877–886. https://doi.org/10.2533/000942905777675318
Chollet M, Prieur D, Böhler R, Belina R, Manara D, The melting behaviour of uranium/neptunium mixed oxides, J. Chem. Thermodynamics, 89 (2015) 27–34. http://dx.doi.org/10.1016/j.jct.2015.04.031
Chen J-L, Kaltsoyannis N, DFT + U study of uranium dioxide and plutonium dioxide with occupation matrix control, J. Phys. Chem. C, 126 (2022) 11426−11435. https://doi.org/10.1021/acs.jpcc.2c03804
Chen J-L, Kaltsoyannis N, DFT + U study of U1-y AnyO2-x (An = Np, Pu, Am and Cm) {111}, {110} and {100} surfaces, Appl. Surf. Sci., 537(5) (2021) 147972. https://doi.org/10.1016/j.apsusc.2020.147972
Mccleskey TM, Bauer E, Jia Q, Burrell AK, et al., Optical band gap of NpO2 and PuO2 from optical absorbance of epitaxial films, J. Appl. Phys., 113(1) (2013) 013515. https://doi.org/10.1063/1.4772595
Chen J-L. Theoretical study of actinide oxides [dissertation]. Manchester (United Kingdom): The University of Manchester; 2020. 246 p.
Cococcioni M, de Gironcoli S, Linear response approach to the calculation of the effective interaction parameters in the LDA+U method, Phys. Rev. B, 71 (2005) 035105. https://doi.org/10.1103/PhysRevB.71.035105
Qiu R, Ao B, Huang L, Effective Coulomb interaction in actinides from linear response approach, Comp. Mater. Sci., 171 (2020) 109270. https://doi.org/10.1016/j.commatsci.2019.109270
Aryasetiawan F, Imada M, Georges A, Kotliar G, Biermann S, Lichtenstein AI, Frequency-dependent local interactions and low-energy effective models from electronic structure calculations, Phys. Rev. B, 70 (2004) 195104. https://doi.org/10.1103/PhysRevB.70.195104
Aryasetiawan F, Karlsson K, Jepsen O, Schönberger U, Calculations of Hubbard U from first-principles, Phys. Rev. B, 74 (2006) 125106. https://doi.org/10.1103/PhysRevB.74.125106
Gunnarsson O, Andersen OK, Jepsen O, Zaanen J, Density-functional calculation of the parameters in the Anderson model: application to Mn in CdTe, Phys. Rev. B, 39 (1989) 1708–1722. https://doi.org/10.1103/PhysRevB.39.1708
Anisimov VI, Gunnarsson O, Density-functional calculation of effective Coulomb interactions in metals, Phys. Rev. B, 43 (1991) 7570–7574. https://doi.org/10.1103/PhysRevB.43.7570
Sato A, Hada M, Abe M, Electron correlation effects on uranium isotope fractionation in U(VI)–U(VI) and U(IV)–U(VI) equilibrium isotopic exchange systems, Phys. Chem. Chem. Phys., 26 (2024) 15301–15315. https://doi.org/10.1039/d4cp01149j
Abe M, Suzuki T, Fuijj Y, Hada M, Hirao K, An ab initio molecular orbital study of the nuclear volume effects in uranium isotope fractionations, J. Chem. Phys., 129 (2008) 164309. https://doi.org/10.1063/1.2992616
van Stralen JNP, Visscher L, Larsen CV, Jensen HJA, First-order MP2 molecular properties in a relativistic framework, Chem. Phys., 311 (2005) 81–95. https://doi.org/10.1016/j.chemphys.2004.10.018
Møller C, Plesset MS, Note on an approximation treatment for many-electron systems, Phys. Rev., 46(7) (1934) 618–622. https://doi.org/10.1103/PhysRev.46.618
Shamov GA, Schreckenbach G, Vo TN, A comparative relativistic DFT and ab initio study on the structure and thermodynamics of the oxofluorides of uranium(IV), (V) and (VI), Chemistry, 13(17) (2007) 4932–4947. https://doi.org/10.1002/chem.200601244
Kovacs A, Molecular oxides of high-valent actinides, Struct. Chem., 31 (2020) 1247–1271. https://doi.org/10.1007/s11224-020-01555-3
Gueneau C, Chartier A, Van Brutzel L, 7.03–Thermodynamic and thermophysical properties of the actinide oxides, Comprehensive Nuclear Materials. 2nd ed., 7 (2020) 111–154. https://doi.org/10.1016/B978-0-12-803581-8.11786-2
Clavaguera-Sarrio C, Vallet V, Maynau D, Marsden CJ, Can density functional methods be used for open-shell actinide molecules? Comparison with multiconfigurational spin-orbit studies, J. Chem. Phys., 121 (2004) 5312–5321. https://doi.org/10.1063/1.1784412
Wen X-D, Martin RL, Scuseria GE, Rudin SP, Batista ER, A screened hybrid DFT study of actinide oxides, nitrides, and carbides, J. Phys. Chem. C, 117(25) (2013) 13122–13128. https://doi.org/10.1021/jp403141t
Luo Z, Qin X, Wan L, Hu W, Yang J, Parallel implementation of large-scale linear scaling density functional theory calculations with numerical atomic orbitals in HONPAS, Front. Chem., 8 (2020) 589910. https://doi.org/10.3389/fchem.2020.589910
Mohr S, Ratcliff LE, Genovese L, Caliste D, et al., Accurate and efficient linear scaling DFT calculations with universal applicability, Phys. Chem. Chem. Phys., 17 (2015) 31360–31370. https://doi.org/10.1039/C5CP00437C
DOI: https://doi.org/10.15826/elmattech.2024.3.044
Copyright (c) 2024 Alexander Y. Galashev

This work is licensed under a Creative Commons Attribution 4.0 International License.